Coal’s Importance for Solar Panel Manufacturing
By Dr. Lars Schernikau
Coal is not the favorite “child” these days. It seems that almost the entire western political world has sworn to send coal to its grave. Not only have the United Nations and the IEA literally declared “war” on coal, but countless political, activist organizations and even leading financial institutions have pledged, if it had to be in their power, to immediately stop the usage of coal.
The reason for all of this is of course this “terrible” chemical element called carbon (number 6 on the periodic table). Please remember though that the same carbon is the 2nd most abundant element in the human body and it is a key building block for all life on Earth. By the way, carbon is not only essential because CO2 is plant food and plants grow best at 1.500 ppm of CO2 in the air (current atmospheric content is 420 ppm), CO2 is also a greenhouse gas, contributing to keeping our Earth temperature temperate and livable.
I have to mention that the prize for keeping Earth livable has to go to water, or better yet, water vapor, the most important and most abundant greenhouse gas. We all understand that increased greenhouse gas concentrations will contribute to slight warming, though only a few of us have learnt – including me only after studying it – that there are so-called saturation levels to consider which means that higher concentrations of any greenhouse gas have less and less impact on temperature changes (the warming impact logarithmically declines).
But today’s blog is not about globally measured temperature changes, its causes and its negative or positive impacts, but about coal and solar.
So why are coal and solar so closely interlinked? Why is it that solar panel manufacturing is impossible without coal? I always thought that coal is “only” important for electricity, contributing to 36% of global power demand, or over 8h of 24h every single day of the year. I always thought that coal is “only” required to produce all steel. Let us have a look at solar panel manufacturing, which is really about silicon production.
The vast majority of all energy required to make solar panels is consumed during silicon production, purification, and wafering. But first let’s talk about purity. 6N pure silicon means 99.9999% purity level, 11N pure silicon means 99.999999999% purity level, you get the point.
You may now have a first glimpse of the chemical and mechanical difficulty of making such a pure metal from a natural product.
In this blog post, you will see how important uninterrupted power supply is, especial for industrial processes such as silicon smelting. Obviously, this power comes from coal in China, and cannot come from wind or solar. Let’s dig deeper.
1. Metallurgical-grade silicon making and high purity quartz (HPQ)
Elemental silicon (Si) is not a naturally available element. Largely unchanged for over 100 years, silicon (Si) is produced by chemically reducing mined high purity quartz (SiO2) using carbon (C) in submerged-arc furnaces. The arc furnaces are each powered by up to 45 megawatts of electricity also to produce the heat required for the processes. As the mix of quartz stone and carbon heats, the carbon reacts with the oxygen in the quartz and forms CO gas, this is called silicon smelting. Consider it like iron ore (Fe2O3) being reduced using coke from coking coal (C) to make iron (Fe).
All simplified
- Iron making: Fe2O3 + 3C + heat => 2Fe + 3CO
- Silicon making (smelting): SiO2 + 2C + heat => Si + 2CO
This means that each ton of silicon roughly releases 5-6 tons of CO2 in this silicon smelting process alone.
High purity quartz sand (HPQ) is the feedstock for metallurgical-grade silicon. It is generally considered that the starting quality of feedstock for solar panels and semi-conductors is 99.95% silicon oxide (SiO2), with only <500 ppm of total impurities. Such HPQ is scarce and needs to be mined, processed, and of course transported before it is ready to be used for smelting (Chemical Research 2023 and Troszak).
The typical processing sequence for high-purity quartz includes:
- (a) pre-treatment, which involves crushing, scrubbing, desliming, screening, and grinding;
- (b) physical separation methods, including radiometric sorting, dense media separation, gravity separation, magnetic–electric separation, and flotation;
- (c) chemical treatments, such as calcination-water quenching and leaching; and
- (d) advanced treatments, encompassing chlorination, roasting and vacuum refining (Zhang et al 2023).
Estimates of the energy and therefore also CO2 footprint of silicon manufacturing diverge widely in the literature or “scientific community”. Though I believe we already understand that global silicon purification and solar panel manufacturing is dominated by China (Figure 1).
2. Carbon sources for silicon making: Coal, petcoke, hardwood
Interesting is that various sources of carbon are used for the silicon smelting process. These carbon sources are derived largely from coal, petcoke (a byproduct of oil refining) and hardwood. Coal, to make coke, is the most important, but this coal must be of special quality, very low ash, high fixed carbon, with specific reactivity (tested using SINTEF tests), and of a specific size. This coal is rather scarce globally, with Colombia playing an important role. For more detail on silicon smelting please also see Troszak’s 2019, Burning coal and trees to make solar panels.
The mining of such coal is not only expensive, because it is scarce and requires large overburden removal, but also the coal processing (washing) requires energy and “wastes” resources. Once washed and ready, only a fraction of the coal consisting of specific sizing, usually 3-12mm can be used in the furnaces used for silicon smelting. The finer material has to be sold at lower values. Furthermore, to maintain the sizing, the coal should be shipped in bulker bags or sea containers so the sizing does not degrade with handling.
You can see why such special coal demands a large premium and a significant amount of energy for mining, processing/upgrading/sizing, and then of course transportation to the smelters (thanks also to Rob Boyd from New Zealand for his valuable input).
Hardwood is a remarkable one. Shredded hardwood must be mixed into the silicon smelter “pot” to allow the reactive gasses to circulate, so that the liquid silicon that forms, can settle to the bottom for tapping, and to allow the resulting CO (and other gasses) to escape the smelter “charge” safely (Troszak 2019). Woodchips provide a large surface area for the chemical reaction to take place more completely and at improved rates.
Hardwood helps to maintain a porous charge, thereby promoting gentle and uniform – instead of violent – gas venting. Woodchips help regulate smelting temperatures to keep the furnace burning smoothly on top, reducing conductivity, promoting deep electrode penetration, reducing dust, and help in preventing bridging, crusting, and agglomeration of the mix (Wartluft 1971).
Of course, aged hardwood trees are required to be burned to make woodchips. Hardwood is biomass that is extracted from nature but those trees, i.e. in the Brazilian Amazon, you may not be surprised, take more than a couple of years to grow.
3. Solar-grade silicon (SoG-Si) making and wafering
For solar panel manufacturing to be complete, more is required. Metallurgical grade silicon (MG-Si) from the smelter, usually of 98% purity, does not meet the purity requirements of the photovoltaic industry, so it must undergo two more energy-intensive processes before it can be made into solar cells and then into panels.
Firstly, the Siemens Process converts metallurgical grade silicon (MG-Si) from the smelter into polycrystalline silicon (called polysilicon) by using an extremely energy intensive process, a high-temperature vapor deposition process (Troszak 2019). The purity requirement for solar grade silicon (SoG-Si) is currently 9-11N (99.999999999%), a factor of 10.000 to 100.000 more pure compared to the 5-6N purity required for solar PV a decade ago and likey the basis for the solar panels on your roof (if you have some). In the Siemens process, silicon is crushed and mixed with hydrochlorous acid (HCl) to create Trichlorosilane gas (SiHCl3). This gas is heated and deposited onto very hot rods of polysilicon (1.150C) while the reaction chambers walls are cooled.
Each batch of polysilicon “rods” takes several days to grow, and a continuous, 24/7 supply of electricity to each reactor is essential to prevent a costly “run abort.” Polysilicon refineries depend on highly reliable conventional power grids, and usually have two incoming high-voltage supply feeds. (Sources Mariutti and Schernikau 2024, unpublished academic paper, Troszak 2019).
Secondly, the Czochralski Process turns the liquid silicon metal from the smelt and doping materials (gallium or phosphorous) into the silicon ingot, a large monocrystal, 20-30 cm diameter and 1-2 m in length. Next, the ingot is sawed into rectangular bricks, which are sliced into wafers using a diamond wire sawing process (Figures 3 and 4). This process requires several days, and uninterrupted 24/7 power supply. An ingot/wafer/cell plant can use more than 100 MWh additional energy per ton of incoming polysilicon, which is about 6 times as much as the original smelting of the silicon from ore.
Estimates of the energy and therefore CO2 footprint of silicon purification and wafering also diverge widely in the literature, mainly due to two reasons. On the one hand, there is no agreement on the estimated energy demand for these core processes. For example, solar grade silicon (SoG-Si) is the most energy-intensive step in the silicon purification process and should best be understood. Yet, SoG-Si inventories report an electricity demand ranging from 50 kWh/kg to 110 kWh/kg, which appears quite low.
On the other hand, secondary and pre-smelting processes are rarely included when considering the definition of an energy footprint, applicable to the average Chinese silicon industry. Currently, reporting used by governments for decision making, tend to be based on best-in-class plants, like in Europe or North America, which is far removed from reality.
4. Finalizing solar panel manufacturing
Once wafers are produced a few more steps are required before we have a ready-made solar panel. All of these steps require a significant amount of energy in addition to the raw materials required to build the factories and machines, the running of processes and operations, and the supply of electricity and heat required to perform these processes.
- Wafer sawing: Silicon “bricks” are sliced into thin wafers for later manufacturing of solar cells
- Solar cell and module production: requiring aluminum, glass, copper, plastic, rare earths, acids, and over 400 chemicals
- Mounting structure supply: requiring aluminum frames, cement foundation, etc.
- Transportation: everything needs to be transported to the point of use i.e. in the US or Germany
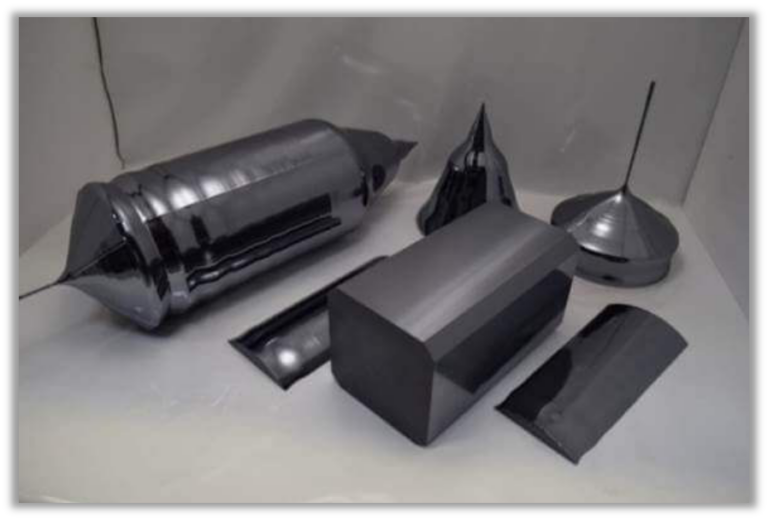